Numerical simulation of quark-gluon plasma formed in the collision of two gold atoms.
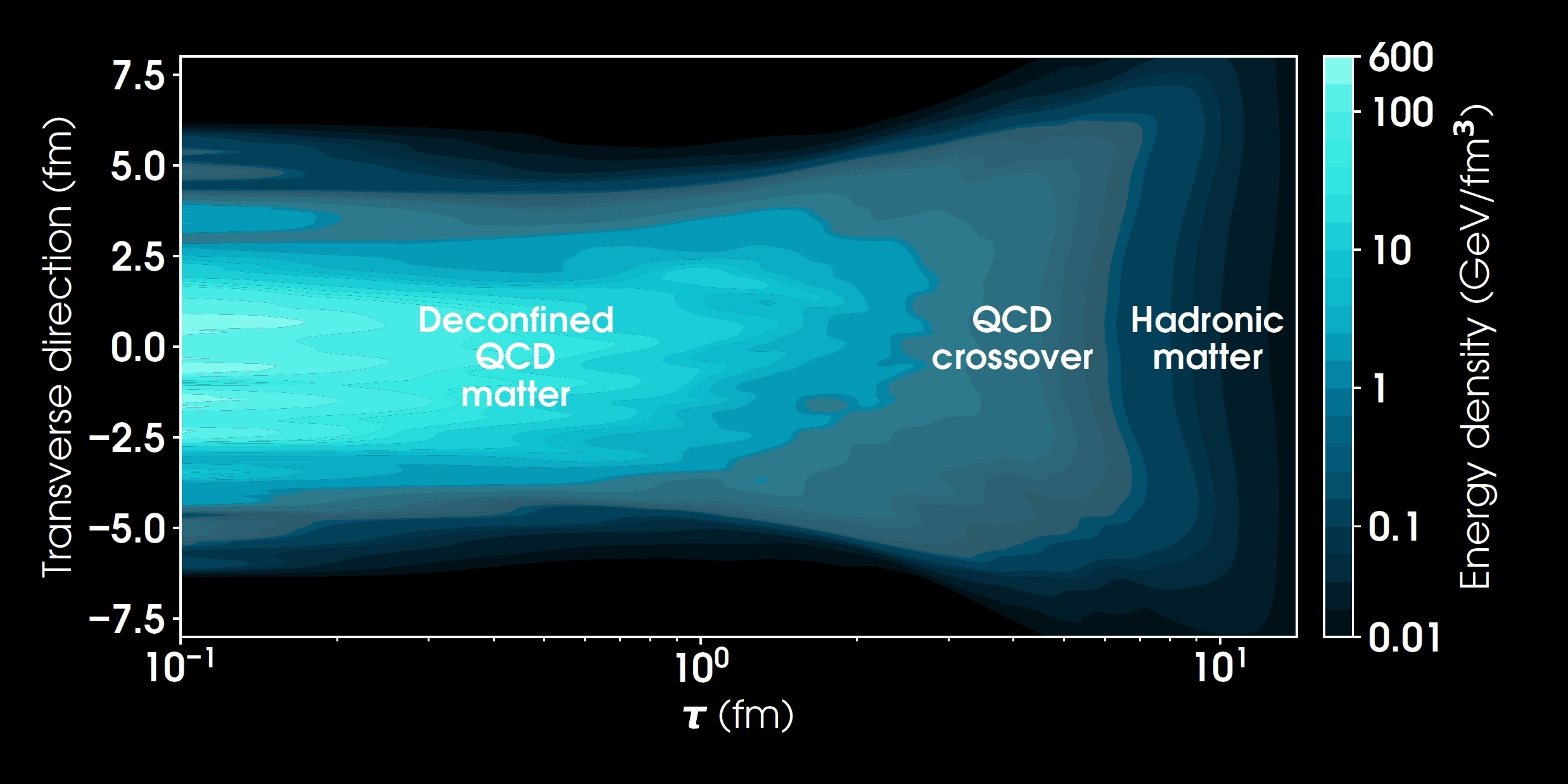
[ jean-francois.paquet@vanderbilt.edu ]
[InspireHEP] [ORCID] [Google Scholar]
Assistant Professor of Physics and Mathematics
Quarks and gluons are fundamental building blocks of matter. Their interactions are governed by Quantum Chromodynamics, one of the core parts of the Standard Model of particle physics.
Quark-gluon plasma can now be produced in laboratory, by colliding large nuclei at velocities close to the speed of light. The two colliders that can achieve this feat are the Relativistic Heavy Ion Collider at Brookhaven National Laboratory (New York) and the Large Hadron Collider at CERN (Switzerland/France).
Quark-gluon plasma is never seen directly: its existence and properties must be inferred from the shower of subatomic particles that hits the detector (image on the right). I study the properties of quark-gluon plasma using multi-stage numerical simulations whose core is relativistic viscous fluid dynamics.
I also use Bayesian inference to quantify the properties of quark-gluon plasma with reliable uncertainties. The specific shear viscosity of quark-gluon plasma is among the smallest ever measured in a liquid (an honour shared with cold atomic gases); it is orders of magnitude smaller than that of liquid helium, for example.
The figure on the left shows a recent constraint on the specific shear viscosity η/s of quark-gluon plasma as a function of the plasma's temperature (the orange band is a Bayesian model average, the bottom panel is the posterior-prior Kullback–Leibler divergence). This result is the product of a multi-institution collaboration, obtained from millions of core-hours of calculations on US supercomputers.
While the viscosity of strongly-coupled quark-gluon plasma is famously difficult to calculate, the long-term objective is to find agreement between measurements of the viscosity and ab initio calculations.
I also study the production of photons by the quark-gluon plasma. Because the quark-gluon plasma produced in nuclear collisions is small, photons can escape with negligible rescattering, and they can be used to probe deep into the hottest and densest parts of the plasma. Recently, I have used analytical solutions of relativistic fluid dynamics (the "Gubser solution") to better understand the relation between the energy spectrum of photons, the temperature of the plasma and the Doppler shift on photons resulting from the plasma's expansion, finding \(T_{\textrm{eff}} \approx T_0/(1+\frac{5}{2} \frac{T_0}{E})\).
The figure on the left shows this relation between the actual maximum temperature of the plasma and the apparent ("effective") temperature extracted from the photon spectrum in different energy ranges.
See also our latest work on the relation between the speed of sound of quark-gluon plasma and the final particles' energy and multiplicity.
(adapted from a topical review I wrote in 2024)
We aim to describe nucleus collisions from the initial impact of the nuclei to the final shower of particles observed by detectors.
The problem is broadly approached piecewise. Nuclear structure properties of heavy nuclei, measured in scattering experiments, can be combined with effective field theory calculations to understand the state of the nuclei shortly after their impact. Many-body quantum field theory is used to study the interaction of energetic quarks and gluons with their lower-energy counterparts.
In certain regimes, the collective motion of strongly-interacting quarks and gluons is described with relativistic fluid dynamics, using an equation of state calculated numerically from lattice quantum field theory. By combining these different theoretical approaches and more, one can obtain a multi-stage model that is a functional description of the successive stages of ultrarelativistic nuclear collisions. Any quantity that is unknown or only partly constrained by theoretical guidance can be considered a model parameter that enters the description of the collision. For example, the shear and bulk viscosities of strongly-coupled nuclear matter are generally parametrized as a function of temperature, and the same can be done for the equation of state.
A large amount of data is available to constrain the model's parameters, a result of the comprehensive heavy-ion-collision program at the Relativistic Heavy Ion Collider and the Large Hadron Collider. The detectors measure the number and momentum distributions of different species of particles produced after each collision, as well as various correlations between these particles.
The final shower of particles fluctuates from one collision to the next, due to the variable degree of overlap of nuclei --- which cannot be controlled --- and the quantum nature of nuclear collisions. Measurements are defined by averaging over a large number of collisions. The variable degree of overlap of the nuclei --- the centrality --- provides a natural parameter that changes the system's size, geometry and density. By further colliding different nuclei species and varying the collision energy of the nuclei, one can probe the system in a large number of different configurations.
The number of measurements available is large, and the type of measurements varies from simple averages (e.g., particle numbers) to intricate correlation observables. The measurements are stochastic, with statistical uncertainties ranging from a fraction of a percent to tens of percent. There are also systematic uncertainties that can be correlated across different data sets, although information about these correlations is often not readily available. On the theory side, multistage models provide a general description of the collisions to compare with these measurements. There is uncertainty in the physics description of the collisions, represented in the literature by different models used to describe the same stage of the collision.
For example, a given stage of the collision can be parametrized with a flexible ansatz, or one can use an ab initio model with fewer parameters but additional physics assumptions.
J.-F. Paquet
Henry Hirvonen
(postdoc)
Teerthal Patel
(postdoc)
Mayank Singh
(postdoc)
Andi Mankolli
(PhD student)
McKenna Sleeth
(PhD student)
Harvir Dhindsa
(PhD candidate)
Joseph Sanders
(PhD candidate)
Gabriel Soares Rocha
(now faculty at Fluminense Federal University in Niterói, Brazil)
Reach out if you are interested in an undergraduate research project: jean-francois.paquet@vanderbilt.edu
PHYS 8010: Particle and Continuum Mechanics
PHYS 8040: Statistical Mechanics
It may be of some historical interest to recall the peculiar circumstances connected with the publication of the famous memoirs of Professor Gibbs, on which his great reputation rests. They were published in the Transactions of the Connecticut Academy of Sciences, which at that time was not connected with Yale University and received no support from it. When Professor Gibbs presented his papers, the academy had no regular publication funds [...] They were expensive to set up, owing to the complex mathematical formulae. Our funds were small. On nearly every occasion, we had to go out and raise a subscription to pay the cost, partly among college men and partly among the business and professional men of New Haven. Long discussions took place as to our ability to print the articles. Two able mathematical professors were on the committee Loomis and Newton. Both protested that they did not understand Gibbs's papers at all. One insisted that no man ever lived who could except Maxwell and he was dead. Yet we all believed that what Gibbs wrote must be of intrinsic value in his branch of science. Therefore we raised the money and printed each paper as it came in.
A.E. Verrill, "How the works of Professor Willard Gibbs were published" (1925) Science
Introduction to Fluid Dynamics (for nuclear collisions)
Too few people have had the chance to watch this series of videos
from 1961: https://web.mit.edu/hml/ncfmf.html
See
for example, Sir G.I. Taylor himself explain [non-relativistic]
fluid dynamics at low Reynolds numbers.
These videos are a reminder of the decades of experimentation it took to
establish the foundations of non-relativistic fluid dynamics.
A complete list of my publications is available on INSPIRE [ INSPIRE profile ], with links to the published
versions and the (free) arXiv versions. Google Scholar is also eerily good at finding
my work.
In the summer of 2018, I wrote a manual for the heavy-ion
hydrodynamic simulation code MUSIC as support
material for lectures I gave at a workshop at the University of São
Paulo. Since then, a new
official version of MUSIC has been published, and I updated
the manual accordingly for this new version: here.
The older
version of MUSIC is still usable, of course; its manual
remains available here.
While it is MUSIC-oriented, the manual should provide a good
overview of the physics behind hydrodynamic simulations of heavy-ion
collisions in general.
We use relativistic viscous fluid
dynamics to describe the spacetime expansion of quark-gluon plasma.
MUSIC is a full 3+1D code developed in McGill that has been widely
used by us and others to study heavy-ion collisions.
The official website is http://www.physics.mcgill.ca/music/.
The latest version of the code is available here: https://github.com/MUSIC-fluid/MUSIC/
A legacy version of the code is hosted on Sourceforce: https://sourceforge.net/projects/music-hydro/.
In 2018, I wrote a manual for the code, which I have updated since
to keep up with changes in the code: https://webhome.phy.duke.edu/~jp401/music_manual/
A few highlights:
Jean-François Paquet
[ jean-francois.paquet@vanderbilt.edu ]